Bubbles!
How many?
Bubbles are a critical component of beer. Much research and money has gone into studying beer bubbles, how to produce them, how to preserve them, and why they are different from the bubbles of other carbonated beverages. We can say much about the bubbles in our homebrew with only a bit of envelope math.
If we put 8.50 lbs (3.86 kg) of malted barley, which is 62% fermentable, and one 1 lbs (0.45 kg) of sugar into a brew, we get approximately 6.25 lbs (2.83 kg) of glucose. 1 lbs of glucose produces about 1.5 lbs of CO2, so 6.25 lbs of glucose produces about 9 lbs of CO2. Air at standard temperature (0°C or 32°F) and pressure (one atmosphere or 101,325 PA) has a density of 1.2 kg/m3, and air is 2/3 as dense as CO2. Meaning that our 9 lbs of CO2 would occupy 2.25 m3 (2,250 L, 80 ft3) in those same conditions.
A typical CO2 bubble has a diameter of 0.5 mm and a volume of 1/15 mm3. Divide 2.25 m3 of CO2 by the volume of a typical bubble, and you get 35 billion (3.5 x 1010) bubbles. This is envelope math, which is admittedly sloppy and doesn't account for variations, so we'll say that a six-gallon batch of homebrew produces between 1.0 x 1010 to 1.0 x 1011 bubbles. We aren't swimming in bubbles whenever we open a beer because most of that CO2 is held in solution under pressure.
That's not all: We place priming sugar in the bottles. 8 oz (226.796 g) of priming sugar in 24 bottles means 1/3 oz per 1 L bottle (about 10 g/L). 10 g of priming sugar produces another 5 g of CO2 inside each bottle, which would take up another 2.5 L if not for being under pressure.
That's just how many bubbles a batch of homebrew produces. We can go into greater detail about different phases of bubble physics: Bubble formation → beading/creaming (forming a head) → disproportionation (big bubbles get bigger and small bubbles get smaller) → drainage (liquid beer falls out of the head).
Formation
Bubbles form around nucleation sites, which are impurities in the beer (rare) or on the surface of the glass (common) that allow for CO2 to come out of solution. Beer "fobs" or foams excessively when there are too many nucleation sites, but simply rinsing the interior of the glass fixes this by washing impurities away or filling in scratches with water. Careful observation of a freshly poured pint of beer reveals that "beer bubble size varies slowly with nucleation site size and is pretty much constant for a given site," (Denny, p. 118), and we can say this with physics after defining some words:
- Nucleation site radius, R
- Beer surface tension, γ
- Beer density, ρ
- The acceleration due to gravity near the earth's surface, g = 9.80 m/sec2
And now:
The mean bubble radius to emerge from a nucleation site, r = (3Rγ/2ρg)1/3
Beading


"Beading" or "creaming" is how foam forms from bubbles rising out of a glass of beer. There are actually two types of foam: "wet foam" consists of spherical bubbles with liquid beer between them and "dry foam" consists of polyhedal bubbles with no liquid between them. Liquid foam slips and slides while dry foam is stiff and sticky.
Let's first define some terms:
- Small interval of time, dt
- Height of dry foam, hd
- Height of wet foam, hw
- Height of liquid beer, H
- Fraction of wet foam that drains into liquid beer, a dt
- Fraction of dry foam that drains away into wet foam as bubbles burst, b dt
- Fraction of wet foam that forms into bubbles that rise into dry foam, c dt
- Factor by which wet foam is less dense than liquid beer, r1 = 0.36 since wet foam must be at least 36% liquid for bubbles to remain spherical
- Factor by which dry foam is less dense than wet foam, r2
- Factor by which dry foam is less dense than liquid beer, r1r2
Now we can come up with three equations that describe the change in beer foam composition over time:
Change in dry foam over time, dhd/dt = c/r2 hw - bhd
Change in wet foam over time, dhw/dt = -(a + b)hw + br2hw
Change in liquid beer over time, dH/dt = ar1hw

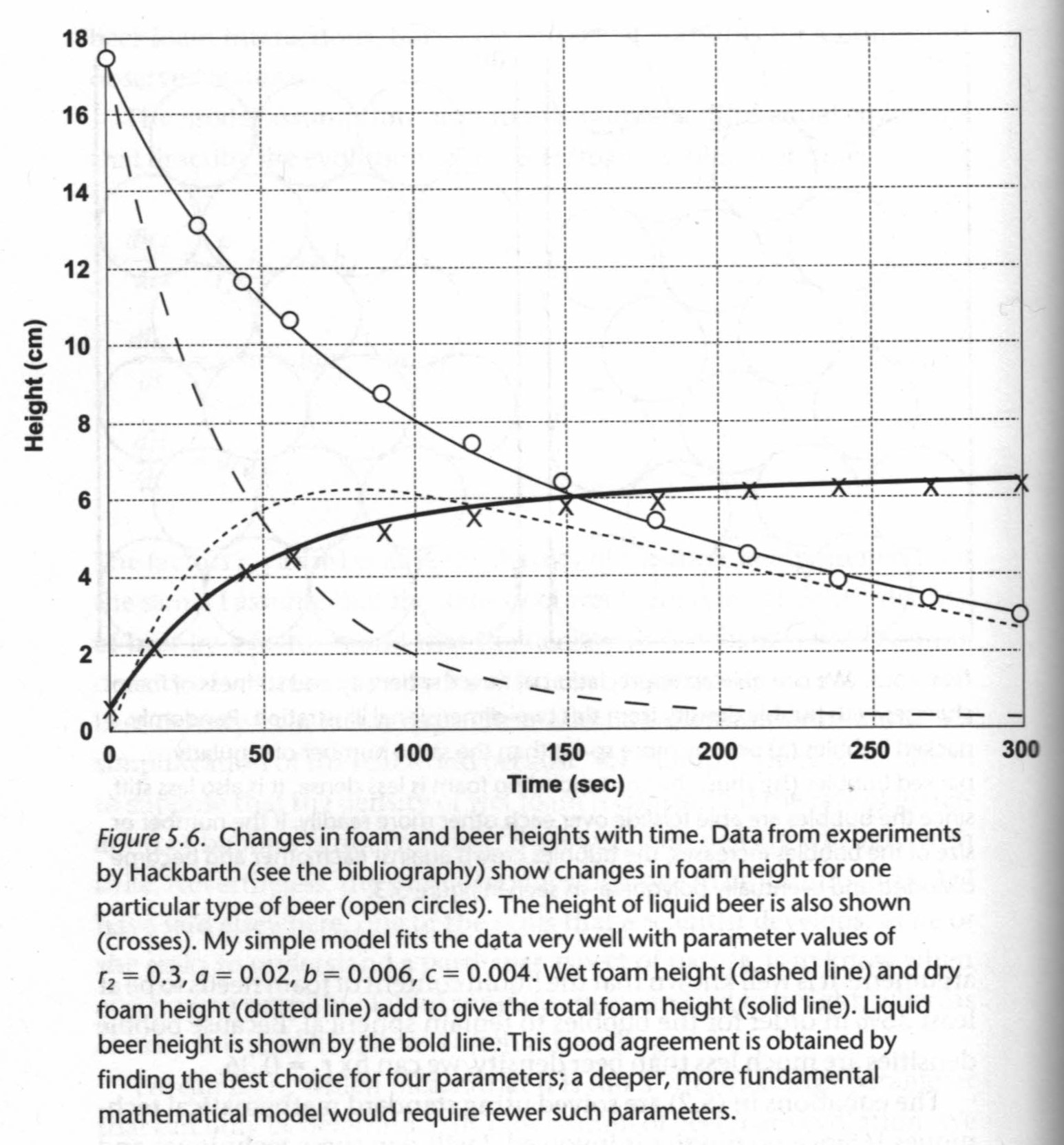
Disproportionation
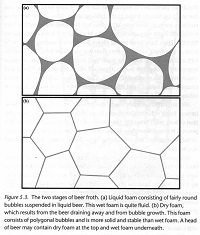
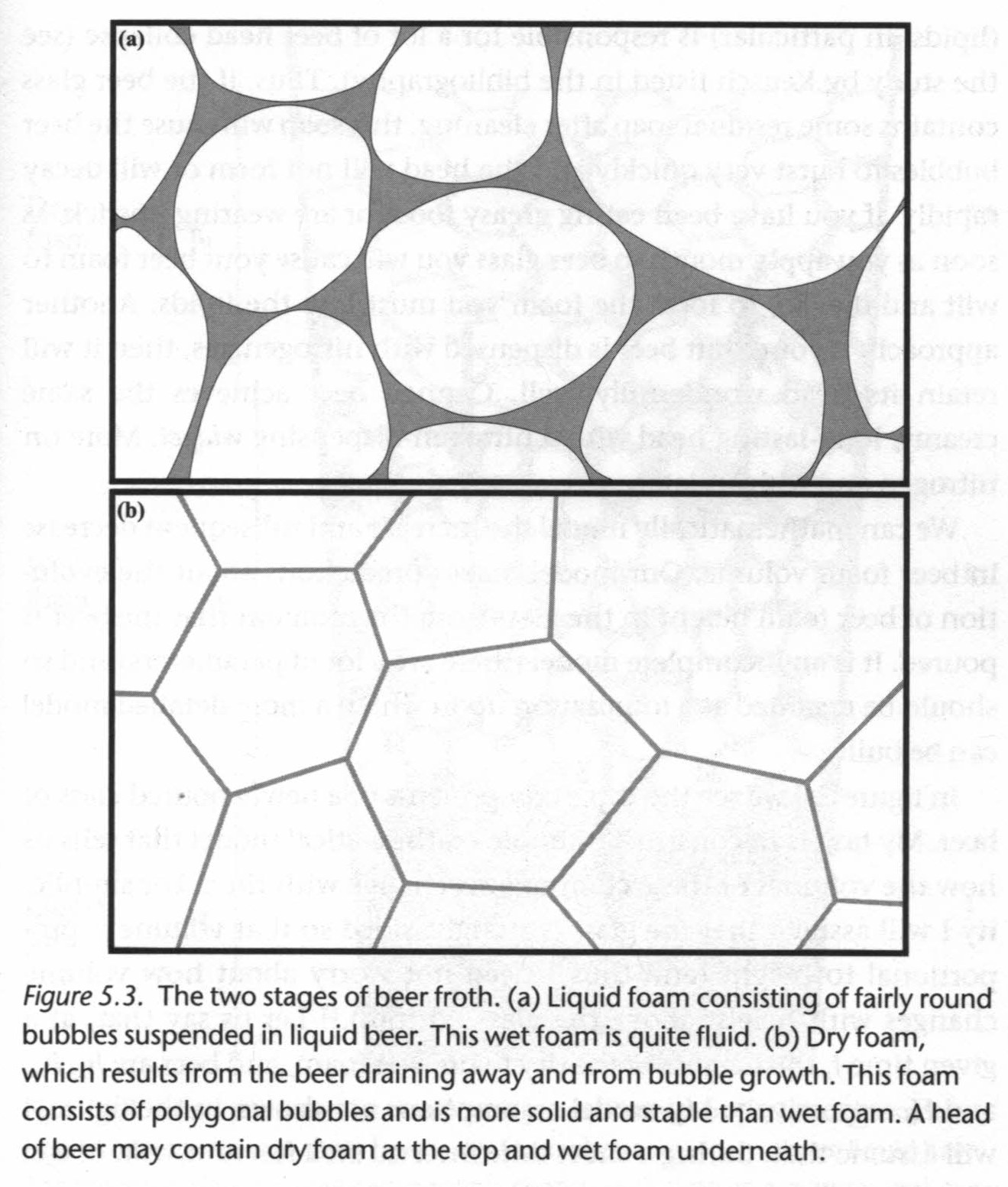
Disproportionation is when large bubbles get larger and small bubbles get smaller as the small bubbles pass their gas to the large bubbles. It is unique to dry foam, where the polyhedral bubbles share boundaries, and happens contrary to what people would likely expect due to the connection between size, surface tension, and pressure. In short, smaller bubbles have higher surface tension and higher pressure. The pressure, not the volume, equalizes between bubbles, so the small bubbles shrink as they lose gas and the large bubbles grow as they gain gas.
If we define a couple more terms, we can make a statement about bubble size:
- Original bubble radius, r0
- Bubble collapse time based on gas pressure, solubility, surface tnesion, and film thickness, t0
And then:
Bubble radius in relation to time, r(t) = r0 sqrt(1 - t/t0)
Beers on a nitrogen tap or with nitrogen in the cans produce more uniform (without disproportionation) foam because nitrogen gas is much less soluble than carbon dioxide. This is also why the head on nitrogen beers lasts longer.
Drainage
Beer bubbles really are special. They last longer and move slower than bubbles in other carbonated beverages, such as soda or champagne, because of surfactants ("surface acting agents" that lower surface tension) that make the beer "sticky" and increases the bubbles' hydrodynamic drag. The bubbles also collect more CO2 and grow as they rise.
We can calculate the speed of a rising bubble relative to the liquid it is in after adding a couple definitions:
- Beer viscosity, η
- Bubble radius, r
And then:
Bubble velocity, v = 2 ρg/9η r2
This isn't the best model as beer bubbles have some unique propeties. Only bubbles smaller than 1/3 mm are spherical; larger bubbles are ellipsoidal. Especially large bubbles (1 mm or greater) have oscillating radii, so the bubbles zizag or spiral as they rise. Beer bubbles do not move with a constant velocity but accelerate as they rise or, as is the case with especially small (1/10 mm or less) bubbles, possibly fall.
Beer bubbles can fall because of the circulation inside the glass. Bubbles rising in the center of the glass, where there is no resistance from the glass walls, drag beer up with them, but the net flow within the glass must be zero otherwise the beer would rise out of the glass unaided. Since it does not do this, the beer on the periphery is actually sinking along the walls. Bubbles on the periphery, which is where most bubbles form due to the availability of nucleation sites, must fight against the current. Especially small bubbles, which are less buoyant and thus slower, rise more slowly or will even fall with the beer.
Antibubbles

The surfactants in beer can also create "antibubbles." Bubbles are little pockets of air within a liquid, so an antibubble is a pocket of liquid within a gas shell within a liquid. This is best demonstrated with a Belgian beer that is poured into another glass of Belgian beer: As the beer pours into the glass, it drags air into the beer, and the air coats droplets of beer instead of dissolving. These air-coated droplets suspended in beer are antibubbles.

Pouring
Now for a bit of practical advice: How to pour a beer correctly. Pouring a beer wrong will not only weaken its smell and flavor, but it will fail to release any of the CO2 contained within the beer, which as we saw above is considerably more than the volume of the bottle itself as it is under pressure, to instead be released inside your stomach.